If we are interested in whether Earth's civilization could ever reach various technological milestones, or long run economic output and populations that Earth could sustain, the terrestrial solar energy resource can provide helpful information. Using efficiencies of the best lab solar cells, covering the earth with solar panel platforms could provide a thousand times as much energy as our civilization currently uses, and more than a hundred times the production of the terrestrial biosphere. Historical experience curve and cost data suggest this energy supply could be much cheaper than current energy prices, although with increasing overhead costs as less desirable areas such as ocean platforms and less sunny lands are used. Energy payback times are already under a year for energy-efficient solar in good locations, and have been falling along with prices, so solar energy can power its own construction, and with advanced robotics and AI might eventually grow at extremely rapid rates.
The total terrestrial solar resource orders of magnitude large than current use
Only a small portion of the solar energy reaching the Earth from the Sun is captured and utilized by human civilization or the rest of the biosphere:
The total power received from the Sun is an overestimate: clouds, atmospheric attenuation, and the angle of the Earth reduce the effective resource by about a factor of four (the day-night cycle reduces average insolation per square meter of Earth's surface but not the total terrestrial resource). 71% of the Earth's surface is covered with ocean, which has about a third of land productivity due to widespread nutrient deficiencies. A third of the land is desert, with more land limited by water or temperature rather than solar resources. Seasonal temperature and precipitation changes further reduce photosynthetic production.
Photovoltaics can be placed in deserts, and even on floating platforms, with a number of projects already completed in calm waters such as reservoirs. The additional costs (one analyst claims inland systems are 10-15+% more expensive)are not normally worthwhile when land area to place the cells is a small portion of costs, but in a world with sufficient demand more fully utilizing the land surface, land savings could offset equipment costs. For oceans with their rougher water and remoteness costs would be still greater, although there has been some development of offshore solar close to island coasts, as in the Maldives, and evidence that the platforms can withstand severe wind and weather.:
Even in times and places of rich biomass production, photosynthesis is inefficient in capturing solar energy. Only about half of the frequencies in sunlight are in the photosynthetically active wavelengths, the process only converts about 30% of energy from those wavelengths, must power the photosynthesis system, suffer photorespiration (especially in C3 plants), and fail to convert all available light (photovoltaic production goes up with insolation, while plants saturate), for peak efficiencies of 3-6% in the most efficient plants (and algae).
By contrast, commercial solar cells have reached efficiencies of over 20%, with the best multi-junction cells in labs (combining multiple photovoltaics to make use of more frequencies and more of the energy in sunlight) in labs of over 47% under concentrated illumination and 39.2% without, using six junctions. From Wikipedia:
A 2011 Department of Energy supported study in Science compared photosynthetic efficiency to photovoltaic-driven electrolysis as means of producing stored chemical energy, concluding that the latter won on energy efficiency. For commercial single-junction solar cells of the day it gave a panel efficiency under ideal conditions of ~18%, 5% loss from changing solar angles over the day, 80% electrolysis efficiency , and 20-30% losses from mismatch between typical solar cell electrical output and electrolysis device requirements, for a total efficiency of 10-11%. That was judged as exceeding peak condition performance of 5-7% for algae in bioreactors, and 4.3% for C4 crops during peak growing season (less than theoretical limits). Over a year with lower production much of the time (no growth periods such as winter, periods when a new crop is small and below peak growth rates, etc) the solar electrolysis system would have a much larger advantage. Producing more complex fuels than hydrogen is also possible, although more difficult, e.g. a 2018 artificial photosynthesis paper reported production of butanol at 8% efficiency
Moving to multi-junction cells with efficiency between those of the best lab demonstrations and thermodynamic limits, automatically redirecting panels through the day, improving on the state-of-the-art in electrolysis, and reducing mismatch look like they could ultimately reach an efficiency of 30%+.
If one covered most of the Earth with such systems (for electricity and chemical energy), that would look to be enough to increase our civilization's energy consumption by more than a thousandfold, to outproduce the biosphere by more than a hundredfold, and to support (at a subsistence level) hundreds of trillions of human bodies (and quadrillions of human brains, or AI computers with similar energy consumption). Such massive deployment would raise other issues, such as changing the Earth's albedo (the terrestrial average is not much off from the solar panel average, but putting dark solar cells in place of more reflective surfaces like ice and snow would increase the Earth's temperature, requiring high albedo offsets elsewhere).
Such absurdly large levels of solar energy production, including in marginal areas such as the open ocean, would only make sense in the context of a combination of vast economic expansion, e.g. because of AI and robotics increasing effective labor more rapidly than humans can reproduce, or over centuries of conventional human reproduction increasing output, reducing solar device costs, and increasing land prices. But they do help to show that there is room for a much larger civilization on Earth.
Projecting solar experience curves suggest prices well below fossil fuels
Much forecasting of the cost of renewable energy technologies is done using experience curve models, in which each doubling of cumulative production leads to a constant percentage improvement in cost, i.e. Wright's law. Nagy et al (2013) used a database of 62 technologies to to see which extrapolation rules best predicted subsequent technological improvement, and found that Wright's law came out ahead of constant proportional improvement with time, economies of scale with instantaneous production levels, time and experrience, experience and scale, and lagged Wright's law.
Across industries, 10-20% cost declines per doubling of cumulative production are typical. For solar the the improvement rate has been especially high, 20% or more:

For more recent data the rate looks more like 30-40%, according to Ramez Naam:
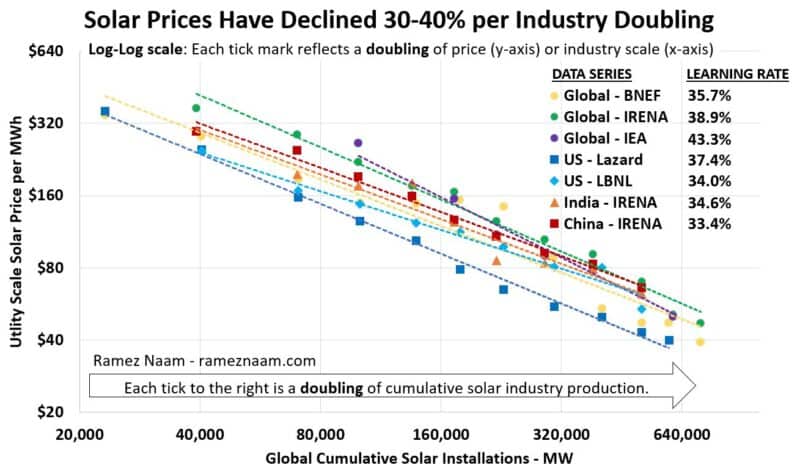
Naam forecasts out using a 30% rate to 19 Terawatts of solar power, 2/3rds of current electricity production, but a smaller share of world energy consumption (of which only about a fifth is electricity)

He argues that such a large rise is possible as economic growth increases total energy demand, transport and industry are electrified, and energy storage and flexible demand such as solar battery charging becomes flexible. If we consider a world with population and economy growing to tap the Earth resources and produce five thousand times as much solar energy, a 20% learning rate would give ~15x cost fall, vs ~75x for a 30% rate. Extremely automated and efficient production processes (paid for by a massive industry), powered by cheap solar, do make massive further price falls conceivable.
Solar needs to be cheaper than other energy sources at scale to pay for its costs of storage, but those are falling as well, although slightly less rapidly (an estimated 18% price fall per cumulative production doubling last year according to BloombergNEF). Timothy B. Lee cites these Bloomberg NEF for these figures:
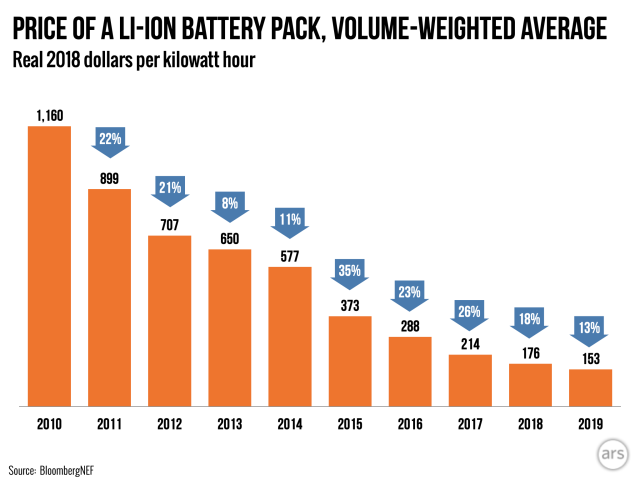
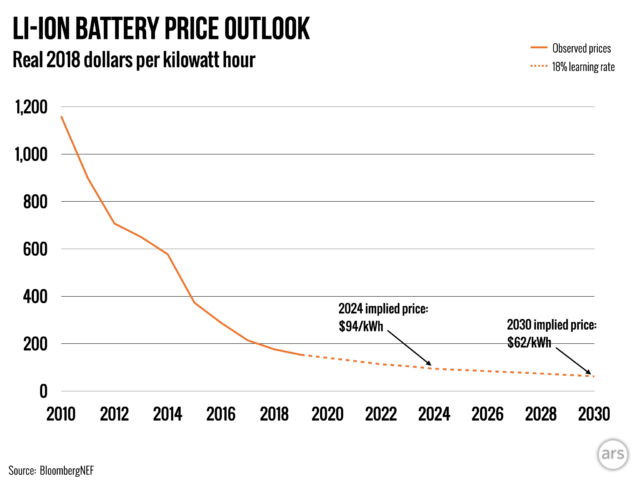
Cheap solar could end a long period of fairly stable real electricity prices
The US. Energy Information Administration (EIA) statistics show that real inflation-adjusted US electricity prices have stayed stable within a factor of 2 for most of a century:
Thus, if solar power costs (including costs of storage and intermittency) continue to decline rapidly past fossil fuel alternatives, the benefit would not only be substitution for fossil fuels and reduced carbon emissions, but large declines in general energy prices. This would be a change from the period of subsidized solar in which higher renewables shares have often increased electricity prices. Overall electricity prices declining in line with solar progress should increase demand and open up new possible uses of energy.
One economic analysis of municipal utility data estimated a long-run (3+ years) price elasticity of local residential electricity demand between -0.29 and -0.39. I.e. a halving of electricity prices might increase consumption in the vicinity of 17%. For sustained regional and global price changes, elasticities would be larger as more energy intensive industries and products are rolled out.
For transport, the use of gasoline by households is quite inelastic in the short-run, as the value of driving for consumers is high relative to gasoline prices, although elasticities are higher in the longer term via investments in fuel efficiency, movement, etc. One reason for this is that fuel consumption in automobiles is paired with the human labor: if it's worth it to spend human wages operating a vehicle, then it's worth spending a substantial fraction of that on gasoline. If self-driving vehicles end the dominance of labor costs in driving, elasticity may increase (e.g. adjusting the frequency and batch size of automated deliveries).
The largest impacts would look to be in (broadly defined) industry. The EIA reports that industry consumes 54% of the world's total delivered energy, with this sectoral breakdown.
Basic chemicals include nitrogen fixation for agriculture, an additional energy input into crops (saving the biological energy expenditures otherwise required for nitrogen fixation via legumes and the like). Much of this energy consumption is in forms that require extra work to use electricity for (batteries to replace gasoline in automobiles, alternative industrial processes), but adjusting for these is a limited constant factor (the analysis is more complicated for intermittency but goes through for with advanced storage).
One state-level analysis of industrial electricity reports a short-run elasticity -0.1, and a long-run elasticity of near -1, but the limited size of the region is likely misleading, since energy-intensive industries (e.g. data centers, aluminium production, etc) migrate to place with low prices. A study of EU demand found a long run price elasticity of industrial electricity use of -0.75 to -1.01, vs -0.53 to -0.56 for residential use.
All told, I'd guess that cutting electricity prices tenfold would increase consumption by at least severalfold. As electrification reaches transport and industry, they would also see increased demand, although heat is cheaper than electricity, so those effects would be lagged.
Declining solar costs can also pay for the overheads of ocean solar platforms, and panels in less sunny regions.
Energy inputs are a significant but minority component of the costs of solar energy, limiting positive feedback loops without radical automation or increased energy demand
Energy costs are a nontrivial component of the costs of producing solar panels and related infrastructure. If advanced solar drives down overall energy prices, then that component of solar costs could also decline, leading to some positive feedback. If energy were the dominant cost of solar, and solar were the main driver of energy prices, then this effect could multiply the impact of solar efficiency improvements by several times.
Where energy is a small proportion of total costs this effect will be small, e.g. if 20% of costs are energy, then solar improvements that double efficiency and cut energy prices in half will yield only an incremental 10% cut in the cost of further solar production, which would flow through to another 1% cut, and converge to only slightly more than the original price fall. That is the situation today.
A recent report by the Fraunhofer Institute for Solar Energy Systems gives energy payback times (the length of time a solar panel needs to operate to generate as much energy as was used in its supply chain) for deployment on the order of a year (varying with technology and solar resource by location) with rapid ongoing improvements:
Financial payback times are severalfold larger than energy payback times, as the costs of labor and capital equipment along the supply chain dominate.
With such low energy payback times, worries about energy cannibalism: solar can power its own expansion (and increased energy costs of mining lower quality ores). The question is more about demand. Solar can expand to replace fossil fuel energy, and increased energy demand with lower prices beyond that, but the learning curve would not predict price falls sufficient to quickly drive demand up to planet-covering levels. For that there would need to be independent increases in demand to pay for the non-energy aspects of production, or radical falls in their costs. A few centuries of human population growth could provide demand increases in line with the population: more energy would be sought to complement the increased labor force.
Advances in AI and automation could allow for increases in the productivity of capital without constraint on complementary human labor, as in various growth models. Low energy payback times would then mean avoiding or delaying energy based bottlenecks. Projecting past trends in energy payback times would suggest they could fall to low months with expansion of solar to a large fraction of current world energy demand, and weeks or even days with extreme terrestrial production scales. Extreme values get some support from looking at biological systems: algae under ideal conditions can reproduce themselves as rapidly as 8 hours.
A robustly robotic/AI economy starting off from a base of less than 10x current world energy consumption could thus have room to grow energy output a couple orders of magnitude, and the possibility of doing so very rapidly.
The total terrestrial solar resource orders of magnitude large than current use
Only a small portion of the solar energy reaching the Earth from the Sun is captured and utilized by human civilization or the rest of the biosphere:
The total power received from the Sun is an overestimate: clouds, atmospheric attenuation, and the angle of the Earth reduce the effective resource by about a factor of four (the day-night cycle reduces average insolation per square meter of Earth's surface but not the total terrestrial resource). 71% of the Earth's surface is covered with ocean, which has about a third of land productivity due to widespread nutrient deficiencies. A third of the land is desert, with more land limited by water or temperature rather than solar resources. Seasonal temperature and precipitation changes further reduce photosynthetic production.
Photovoltaics can be placed in deserts, and even on floating platforms, with a number of projects already completed in calm waters such as reservoirs. The additional costs (one analyst claims inland systems are 10-15+% more expensive)are not normally worthwhile when land area to place the cells is a small portion of costs, but in a world with sufficient demand more fully utilizing the land surface, land savings could offset equipment costs. For oceans with their rougher water and remoteness costs would be still greater, although there has been some development of offshore solar close to island coasts, as in the Maldives, and evidence that the platforms can withstand severe wind and weather.:
Even in times and places of rich biomass production, photosynthesis is inefficient in capturing solar energy. Only about half of the frequencies in sunlight are in the photosynthetically active wavelengths, the process only converts about 30% of energy from those wavelengths, must power the photosynthesis system, suffer photorespiration (especially in C3 plants), and fail to convert all available light (photovoltaic production goes up with insolation, while plants saturate), for peak efficiencies of 3-6% in the most efficient plants (and algae).
By contrast, commercial solar cells have reached efficiencies of over 20%, with the best multi-junction cells in labs (combining multiple photovoltaics to make use of more frequencies and more of the energy in sunlight) in labs of over 47% under concentrated illumination and 39.2% without, using six junctions. From Wikipedia:
The theoretical efficiency of MJ solar cells is 86.8% for an infinite number of pn junctions,[14] implying that more junctions increase efficiency. The maximum theoretical efficiency is 37, 50, 56, 72% for 1, 2, 3, 36 pn junctions, respectively, with the number of junctions increasing exponentially to achieve equal efficiency increments.[24]Additional losses from angles and reflection reduce those limits further. The paper setting the current record claimed that further improvements to their design "could realistically enable efficiences over 50%."
A 2011 Department of Energy supported study in Science compared photosynthetic efficiency to photovoltaic-driven electrolysis as means of producing stored chemical energy, concluding that the latter won on energy efficiency. For commercial single-junction solar cells of the day it gave a panel efficiency under ideal conditions of ~18%, 5% loss from changing solar angles over the day, 80% electrolysis efficiency , and 20-30% losses from mismatch between typical solar cell electrical output and electrolysis device requirements, for a total efficiency of 10-11%. That was judged as exceeding peak condition performance of 5-7% for algae in bioreactors, and 4.3% for C4 crops during peak growing season (less than theoretical limits). Over a year with lower production much of the time (no growth periods such as winter, periods when a new crop is small and below peak growth rates, etc) the solar electrolysis system would have a much larger advantage. Producing more complex fuels than hydrogen is also possible, although more difficult, e.g. a 2018 artificial photosynthesis paper reported production of butanol at 8% efficiency
Moving to multi-junction cells with efficiency between those of the best lab demonstrations and thermodynamic limits, automatically redirecting panels through the day, improving on the state-of-the-art in electrolysis, and reducing mismatch look like they could ultimately reach an efficiency of 30%+.
If one covered most of the Earth with such systems (for electricity and chemical energy), that would look to be enough to increase our civilization's energy consumption by more than a thousandfold, to outproduce the biosphere by more than a hundredfold, and to support (at a subsistence level) hundreds of trillions of human bodies (and quadrillions of human brains, or AI computers with similar energy consumption). Such massive deployment would raise other issues, such as changing the Earth's albedo (the terrestrial average is not much off from the solar panel average, but putting dark solar cells in place of more reflective surfaces like ice and snow would increase the Earth's temperature, requiring high albedo offsets elsewhere).
Such absurdly large levels of solar energy production, including in marginal areas such as the open ocean, would only make sense in the context of a combination of vast economic expansion, e.g. because of AI and robotics increasing effective labor more rapidly than humans can reproduce, or over centuries of conventional human reproduction increasing output, reducing solar device costs, and increasing land prices. But they do help to show that there is room for a much larger civilization on Earth.
Projecting solar experience curves suggest prices well below fossil fuels
Much forecasting of the cost of renewable energy technologies is done using experience curve models, in which each doubling of cumulative production leads to a constant percentage improvement in cost, i.e. Wright's law. Nagy et al (2013) used a database of 62 technologies to to see which extrapolation rules best predicted subsequent technological improvement, and found that Wright's law came out ahead of constant proportional improvement with time, economies of scale with instantaneous production levels, time and experrience, experience and scale, and lagged Wright's law.
Across industries, 10-20% cost declines per doubling of cumulative production are typical. For solar the the improvement rate has been especially high, 20% or more:

For more recent data the rate looks more like 30-40%, according to Ramez Naam:
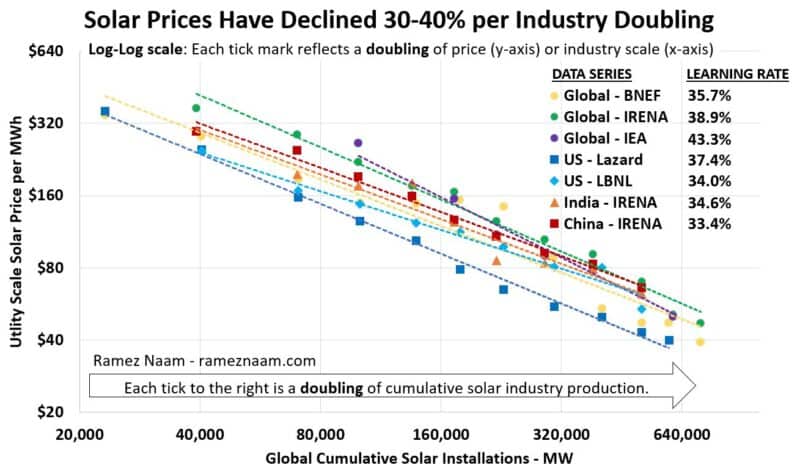
Naam forecasts out using a 30% rate to 19 Terawatts of solar power, 2/3rds of current electricity production, but a smaller share of world energy consumption (of which only about a fifth is electricity)

He argues that such a large rise is possible as economic growth increases total energy demand, transport and industry are electrified, and energy storage and flexible demand such as solar battery charging becomes flexible. If we consider a world with population and economy growing to tap the Earth resources and produce five thousand times as much solar energy, a 20% learning rate would give ~15x cost fall, vs ~75x for a 30% rate. Extremely automated and efficient production processes (paid for by a massive industry), powered by cheap solar, do make massive further price falls conceivable.
Solar needs to be cheaper than other energy sources at scale to pay for its costs of storage, but those are falling as well, although slightly less rapidly (an estimated 18% price fall per cumulative production doubling last year according to BloombergNEF). Timothy B. Lee cites these Bloomberg NEF for these figures:
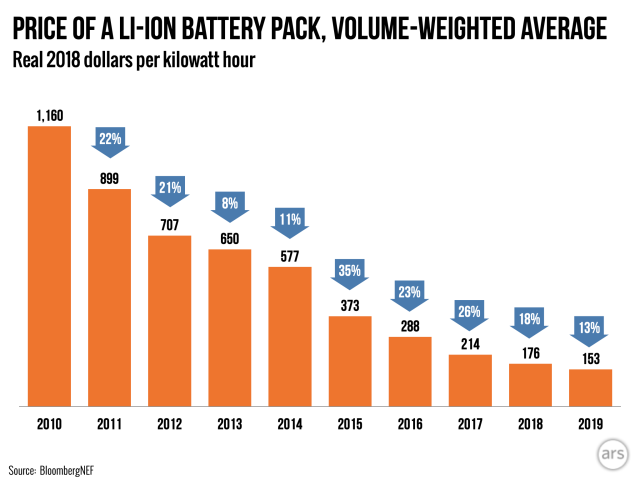
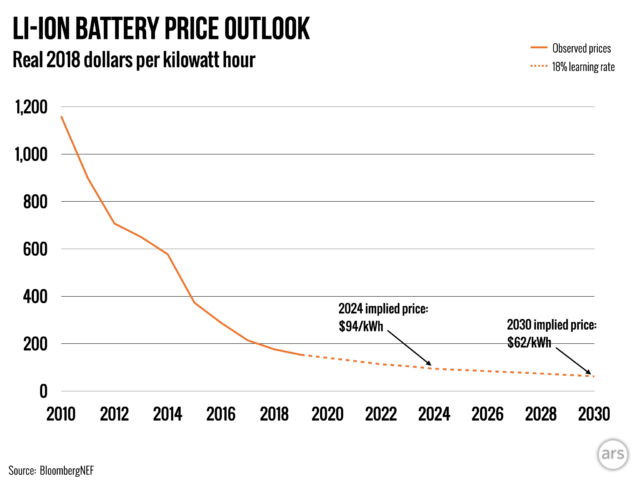
Cheap solar could end a long period of fairly stable real electricity prices
The US. Energy Information Administration (EIA) statistics show that real inflation-adjusted US electricity prices have stayed stable within a factor of 2 for most of a century:
Thus, if solar power costs (including costs of storage and intermittency) continue to decline rapidly past fossil fuel alternatives, the benefit would not only be substitution for fossil fuels and reduced carbon emissions, but large declines in general energy prices. This would be a change from the period of subsidized solar in which higher renewables shares have often increased electricity prices. Overall electricity prices declining in line with solar progress should increase demand and open up new possible uses of energy.
One economic analysis of municipal utility data estimated a long-run (3+ years) price elasticity of local residential electricity demand between -0.29 and -0.39. I.e. a halving of electricity prices might increase consumption in the vicinity of 17%. For sustained regional and global price changes, elasticities would be larger as more energy intensive industries and products are rolled out.
For transport, the use of gasoline by households is quite inelastic in the short-run, as the value of driving for consumers is high relative to gasoline prices, although elasticities are higher in the longer term via investments in fuel efficiency, movement, etc. One reason for this is that fuel consumption in automobiles is paired with the human labor: if it's worth it to spend human wages operating a vehicle, then it's worth spending a substantial fraction of that on gasoline. If self-driving vehicles end the dominance of labor costs in driving, elasticity may increase (e.g. adjusting the frequency and batch size of automated deliveries).
The largest impacts would look to be in (broadly defined) industry. The EIA reports that industry consumes 54% of the world's total delivered energy, with this sectoral breakdown.
Basic chemicals include nitrogen fixation for agriculture, an additional energy input into crops (saving the biological energy expenditures otherwise required for nitrogen fixation via legumes and the like). Much of this energy consumption is in forms that require extra work to use electricity for (batteries to replace gasoline in automobiles, alternative industrial processes), but adjusting for these is a limited constant factor (the analysis is more complicated for intermittency but goes through for with advanced storage).
One state-level analysis of industrial electricity reports a short-run elasticity -0.1, and a long-run elasticity of near -1, but the limited size of the region is likely misleading, since energy-intensive industries (e.g. data centers, aluminium production, etc) migrate to place with low prices. A study of EU demand found a long run price elasticity of industrial electricity use of -0.75 to -1.01, vs -0.53 to -0.56 for residential use.
All told, I'd guess that cutting electricity prices tenfold would increase consumption by at least severalfold. As electrification reaches transport and industry, they would also see increased demand, although heat is cheaper than electricity, so those effects would be lagged.
Declining solar costs can also pay for the overheads of ocean solar platforms, and panels in less sunny regions.
Energy inputs are a significant but minority component of the costs of solar energy, limiting positive feedback loops without radical automation or increased energy demand
Energy costs are a nontrivial component of the costs of producing solar panels and related infrastructure. If advanced solar drives down overall energy prices, then that component of solar costs could also decline, leading to some positive feedback. If energy were the dominant cost of solar, and solar were the main driver of energy prices, then this effect could multiply the impact of solar efficiency improvements by several times.
Where energy is a small proportion of total costs this effect will be small, e.g. if 20% of costs are energy, then solar improvements that double efficiency and cut energy prices in half will yield only an incremental 10% cut in the cost of further solar production, which would flow through to another 1% cut, and converge to only slightly more than the original price fall. That is the situation today.
A recent report by the Fraunhofer Institute for Solar Energy Systems gives energy payback times (the length of time a solar panel needs to operate to generate as much energy as was used in its supply chain) for deployment on the order of a year (varying with technology and solar resource by location) with rapid ongoing improvements:
Financial payback times are severalfold larger than energy payback times, as the costs of labor and capital equipment along the supply chain dominate.
With such low energy payback times, worries about energy cannibalism: solar can power its own expansion (and increased energy costs of mining lower quality ores). The question is more about demand. Solar can expand to replace fossil fuel energy, and increased energy demand with lower prices beyond that, but the learning curve would not predict price falls sufficient to quickly drive demand up to planet-covering levels. For that there would need to be independent increases in demand to pay for the non-energy aspects of production, or radical falls in their costs. A few centuries of human population growth could provide demand increases in line with the population: more energy would be sought to complement the increased labor force.
Advances in AI and automation could allow for increases in the productivity of capital without constraint on complementary human labor, as in various growth models. Low energy payback times would then mean avoiding or delaying energy based bottlenecks. Projecting past trends in energy payback times would suggest they could fall to low months with expansion of solar to a large fraction of current world energy demand, and weeks or even days with extreme terrestrial production scales. Extreme values get some support from looking at biological systems: algae under ideal conditions can reproduce themselves as rapidly as 8 hours.
A robustly robotic/AI economy starting off from a base of less than 10x current world energy consumption could thus have room to grow energy output a couple orders of magnitude, and the possibility of doing so very rapidly.
No comments:
Post a Comment